Theatrical performers in the second half of the 19th century were well and truly in the limelight.The lighting system at the time involved passing three types of burning gases over a heated chunk of limestone until it glowed bright yellow. The light produced was then focused through lenses and mirrors, and directed onto the stage. Not only was this system extremely complicated, it also had the unfortunate habit of burning theatres down.
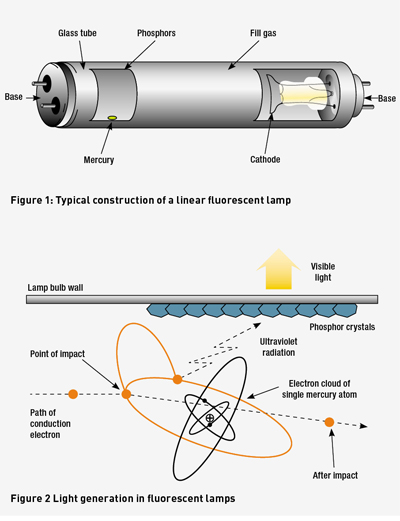
Figures 1 and 2
Phosphorescence and modern lights
Thankfully we've come quite a way from the days of the limelight system. Modern fluorescent lighting systems use the phenomenon of phosphorescence, which is not too unlike the limelight effect. The first fluorescent lamp was patented more than 100 years ago by American inventor Peter Cooper Hewitt.
Cooper Hewitt’s low-pressure mercury arc lamp is the direct parent of today’s fluorescent lights. These lamps are classified as gas-discharge types because they generate light through the excitation of gases by passing an electric current through them. The lamp shown in Figure 1 is generally how all fluorescent lamps are built, whether they're compact fluorescent lamps (CFLs) or linear (LFL) types. All gas discharge lamps have an anode and a cathode.
Anodes and cathodes
Electrons always depart the cathode and impact the anode after travelling through the gas lamp, completing the electrical circuit. However, when alternating current is used, the cathode and anode rapidly switch roles (50 times per second, in Australia). When alternating current is used, the electrical conductors at both ends of the tube are called cathodes.
Each of these cathodes is made of coiled tungsten and is coated with a layer of barium, strontium and calcium oxides chosen to have a low thermionic emission temperature. This allows the cathodes to release more electrons into the gas at lower temperatures, obviating the need for much pre-heating.
The gas filling the tube is largely made up of low-pressure mercury vapour with a mixture of argon, xenon, neon or krypton. Light-emitting phosphors are applied as a paint-like coating to the inside of the tube. This coating is largely responsible for generating the light we see from a fluorescent lamp.
Interestingly, there are trace amounts of mercury beads along the inside wall of the tube when the lamp is cold. During the arcing process, these quickly vaporise and provide the source of short-wave ultraviolet radiation (UV), which is absorbed by the phosphor coating to produce visible light.
Emission of light as photons
The amount of mercury is minuscule and not a cause for concern, but it should be disposed of appropriately if the lamp is damaged. So, the cathodes emit electrons into the gas, and these incident electrons collide with mercury atoms. As a result, kinetic energy is transferred to the atom’s outer electron, causing it to temporarily jump to a higher energy level (or energy band) around the atom.
As this higher energy band is unstable, the mercury atom will emit a UV photon as the atom’s electron reverts to a stable lower energy band. Most of the photons that are released in this way are in the UV region of the spectrum and cannot be seen by the human eye.
UV photons are then absorbed by electrons in the atoms of the lamp’s interior fluorescent coating, causing a similar energy jump, then drop, with the emission of a further photon. The chemicals used in making up the phosphor are chosen so that these emitted photons are in the visible spectrum.
When the light is turned on, the electric current heats up the cathode enough for it to emit electrons. These electrons collide with and ionise noble gas atoms inside the lamp, surrounding the filament to form plasma by the process of impact ionisation. As a result of avalanche ionisation the conductivity of the gas rapidly rises, allowing higher current to flow through the lamp.
The spectrum of light emitted from a fluorescent lamp is the combination of light directly emitted by the mercury vapour and by the phosphorescent coating. Nowadays, this internal coating is based on a tri-phosphate mixture of terbium and europium ions to produce light with more natural colour-rendering properties.
Wavelengths in the visible spectrum
Figure 4 shows the dominant lighting wavelengths that are emitted by this particular fluorescent light source, having a correlated colour temperature in the range of 4,100 Kelvin. Of the wavelengths shown, peaks at indices 2, 4, 5 and 12 appear to dominate the spectrum in terms of intensity.
The blue (index 2) and green (4,5) wavelengths are likely to be generated by the mercury gas vapour and terbium atoms in the doped phosphor coating. The orange-red wavelengths (index 12) are likely to be generated by the europium atoms in the doped phosphor coating.
The reason this light tends to be more acceptable to the human eye is because it has colours in the blue and green spectrum, to which the cones in our eyes are more sensitive. It also has some red, which tends to more closely resemble the colours present in daylight (red, green and blue), with a strong emphasis on blue.
External current regulation and ballasts
Fluorescent lighting systems need some form of external current regulation to prevent them from self-destructing. Unlike incandescent lamps, if left unregulated the current in a fluorescent lamp would continue to grow until the lamp destroyed itself due to the avalanche ionisation effect described earlier.
In addition to current regulation, the lamp also needs a device that starts the arcing effect. Fortunately, the ballast performs all of these functions, and more. All fluorescent lamps need ballasts to work. A ballast is basically a device that stops the flow of current through the lamp from getting out of control.
Older magnetic ballasts employ wire-wound iron cores and operate the lamps at mains frequency. Newer electronic ballasts perform the same function using electronics at much higher frequencies than 50Hz, at approximately 20kHz and above. This is done to improve efficiency in terms of power consumption and light output.
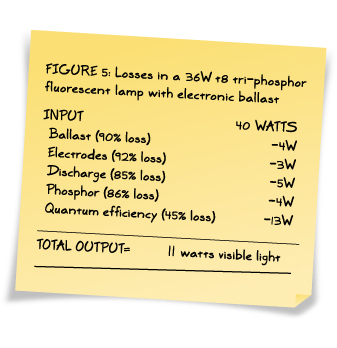
Figure 5
Nevertheless, the fluorescent system is still not perfect. Incandescent lamps convert about 5% of their power into visible light (95% into heat and infra-red radiation), and fluorescent lighting converts about 22% into visible light.
The reasons for this sort of efficiency (or inefficiency) can be described by examining typical losses found in a 36W T8 fluorescent lamp as shown in Figure 5. The ballast losses in magnetic ballasts can be as high as 25% of lamp power, compared with 10% in electronic equivalents. The cathodes also consume power (8% in this example), in that they need to be heated.
Some of the energy in the mercury vapour column is dissipated, with only 85% being converted to short wave UV and visible light. The phosphor typically has a quantum efficiency of 86%. That means that of the 100 incident UV photons that it receives, it converts only 86 of these to visible light photons.
Efficiency and longevity
One of the main reasons for fluorescent and compact fluorescent lighting sources becoming the lamp of choice for consumers is legislative change, with the recent phasing out of incandescent GLS lamps. This was largely on account of the inefficiency outlined above. In stark contrast to the luminous efficacy (the amount of light generated per unit of power applied) of about 13 lumens/watt for a 100W 240V GLS lamp, a typical 23W CFL sits around 60 lumens/watt. That’s four to five times more efficient.
As a rule of thumb, it is probably safe to multiply the power rating of a typical CFL by a factor of five to determine its incandescent power output equivalent. Fluorescent lamps have come a long way over the past few years. Several colour temperatures are available, ranging from warm white (3,000K to 3,500K), cool white (4,100K to 4,200K), full spectrum (4,800K to 5,500K) and daylight (6,000K to 7,000K).
Electronic ballasts have introduced further operational efficiencies and are rapidly becoming more affordable. These ballasts make dimming possible with protocols such as digital addressable lighting interface, allowing feedback to the facility operator for smoother maintenance and more cost-effective operation.
Even though fluorescent lighting is still not as efficient as LED lighting, it is here to stay because of its many benefits – not the least of which is the cost of ownership over time, in addition to energy efficiency.
On a ‘lighter’ note, had our thespian friends of old known about this lighting source, imagine the majestic theatres we could have been enjoying to this day.